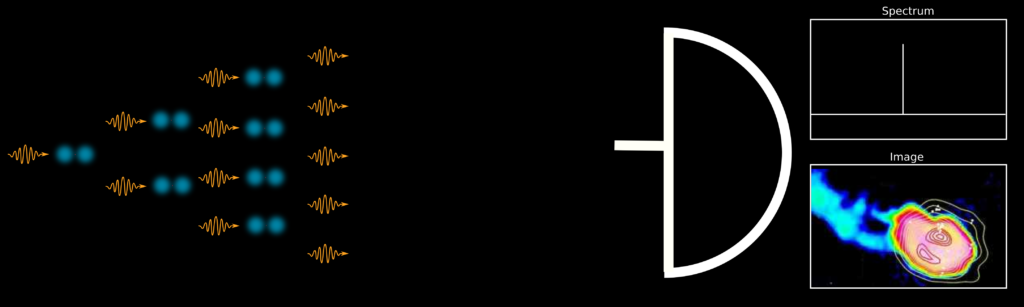
What are astrophysical masers and megamasers?
Astrophysical masers (also known as cosmic masers) and megamasers are masers that naturally occur in space.
Masers are objects that emit radiation amplified by stimulated emission. Their name is an acronym which stands for Microwave Amplification by Stimulated Emission of Radiation.
Astrophysical masers form due to certain densities, temperatures, and the ability of the material to amplify radiation. This means we can use them to study the chemical compositions and the physical conditions of the object producing the maser.
The brightness of cosmic masers along with their single frequency and small sizes also makes them useful in knowing how different parts of an object move.
An example of this are accretion disks around supermassive blackholes that create megamasers. Megamasers are astrophysical masers that are hundreds of millions of times brighter than normal cosmic masers. Such cosmic masers are often around active galactic nuclei and massive star forming regions.
Megamasers have allowed us to determine the masses of supermassive black holes, the size of their accretion disks, and how far away they are. This has allowed us to use such objects to confirm the value of the Hubble constant.
The role of stimulated emission in cosmic masers
Electrons in atoms, molecules, or ions can exist in different energy states. An electron jumps to an excited state when it absorbs a photon with an energy equal to the difference between the excited state and the ground state.
Collisions between atoms can also cause their electrons to jump to excited states.
In the excited state, the electron is unstable and quickly drops to the ground state releasing a photon. This photon has the same energy and frequency as the original photon. This is spontaneous emission.
For stimulated emission to occur, an electron must already be in an excited state when the photon collides with it. The photon must have an energy equal to the difference between the excited state and ground state.
When this photon collides with the electron, it causes the electron to drop to the ground state releasing a second photon. This second photon has the same energy and frequency as the first photon.
For electrons to remain in excited states long enough to create masers, the excited state has to be a metastable state. Metastable states are where electrons can exist for much longer compared to normal excited states.
In clouds of atoms, molecules, and ions that turn into masers, electrons are first energized to normal excited states. They then drop to these metastable states where they can exist long enough for a photon to cause stimulated emission.
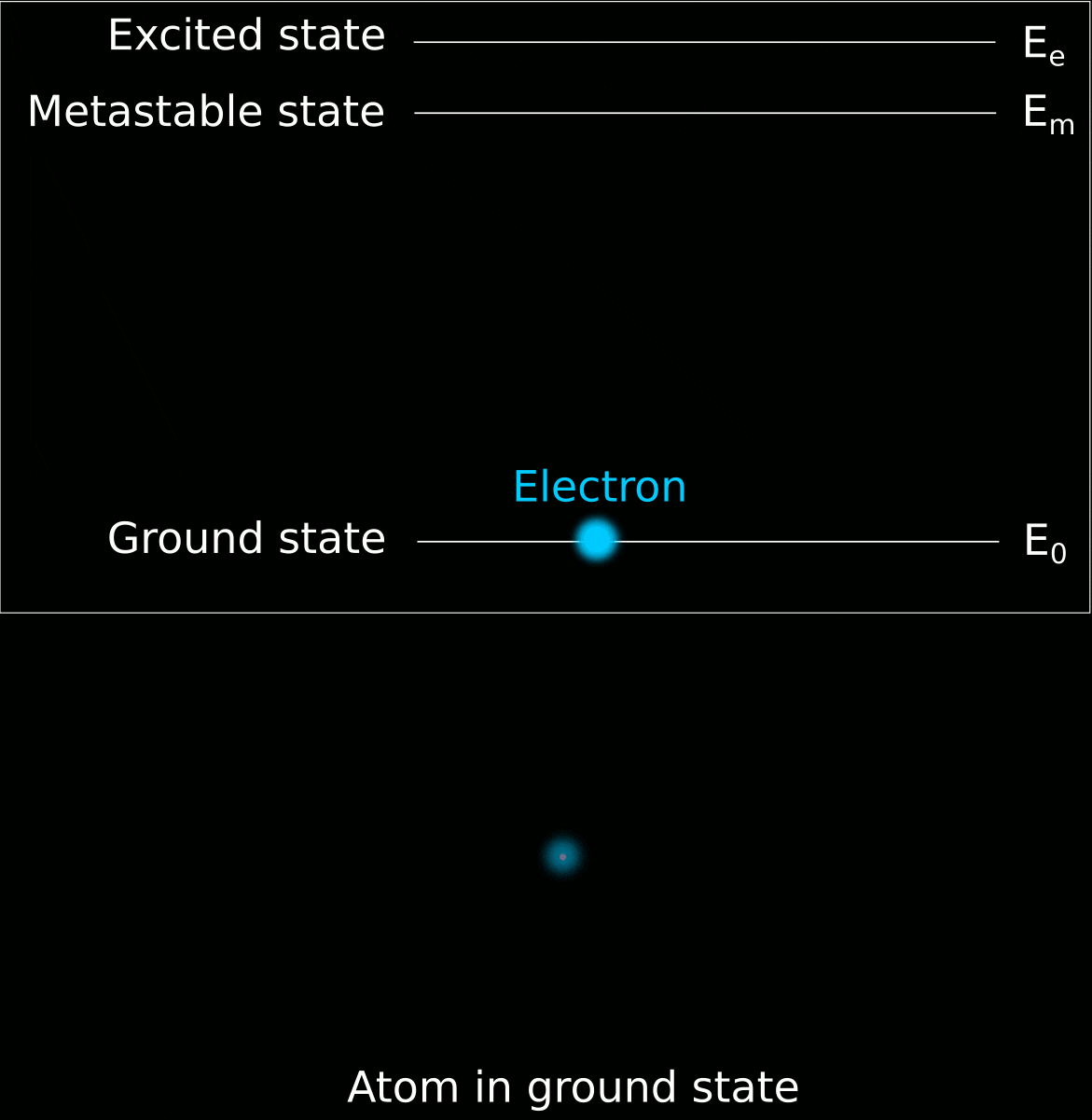
The role of amplification in cosmic masers
A photon created by stimulated emission not only has the same frequency as the original photon. It is also in phase and travels in the same direction. Both photons are therefore coherent. Therefore, the resulting maser radiation is highly amplified.
This sounds very similar to lasers. In fact, they are pretty much the same thing. The only difference is that masers are microwaves. Lasers on the other hand can be visible light, infrared, ultraviolet, or even X-rays.
Masers only form when there is a population inversion. This is when more atoms are in the excited state than the ground state. When this happens, photons are more likely to hit an already excited atom rather than a ground state atom and cause stimulated emission. In this way, the maser radiation gets amplified exponentially.
A cloud of gas that is in thermal equilibrium (i.e. no net flow of heat energy going in or coming out) has more atoms in the ground state than in the excited state. That gas cloud needs an external energy source to achieve population inversion.
We call energy sources that create masers, pumping mechanisms.

Where to find astrophysical masers?
Space has many things that produce lots of energy. In the presence of gas clouds, these things could act as pumping mechanisms needed to create astrophysical masers. Such things can be radiation from stars, collisions between particles at high temperatures, or interactions with magnetic fields.
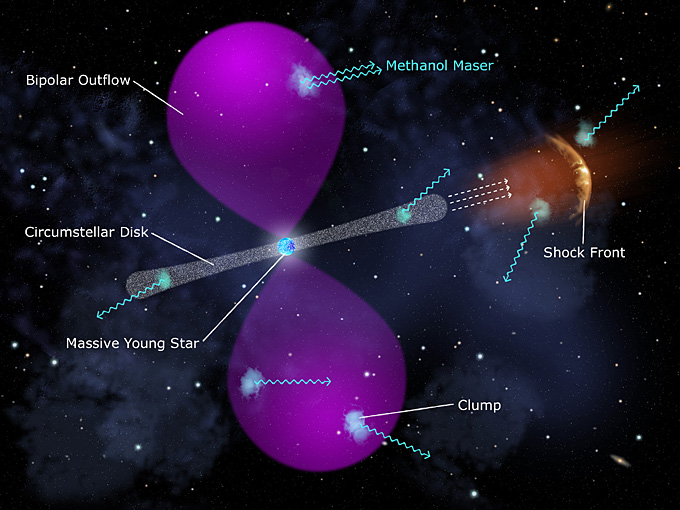
Astrophysical masers were first detected by Weaver et. al. 1965. These masers were located inside molecular clouds and where created by energized hydroxyl molecules. Since then, cosmic masers have been found in a variety of objects.
In space, water (H2O), silicon monoxide (SiO), and hydroxyl (OH) often as part of methanol (CH3OH) are responsible for most maser emissions. Other compounds including ammonia (NH3), formaldehyde (CH2O), methane (CH4) and cyanide (CN-X) can also create astrophysical masers but much less often.
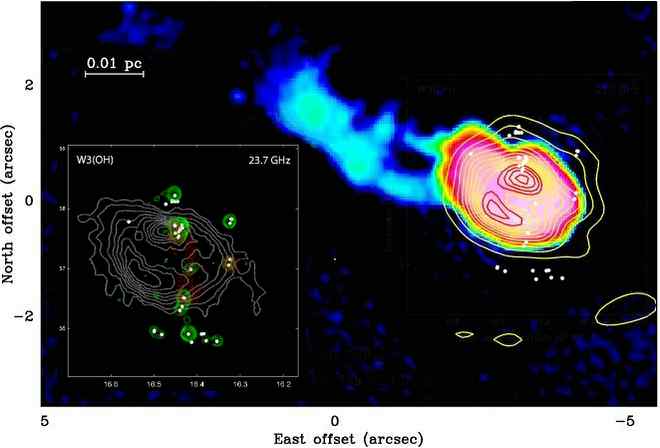
The importance of astrophysical masers in astronomy
As previously said, maser radiation is highly amplified, it has one frequency, and is in phase. This means the light has a distinct and narrow predictable spectrum. A very important feature which allows the detection of red and blue shifts caused by the velocity of the maser.
These properties along with the fact that astrophysical masers are generally small and have high luminosities make them very useful for figuring out the structure and velocities of the objects that they are apart of.
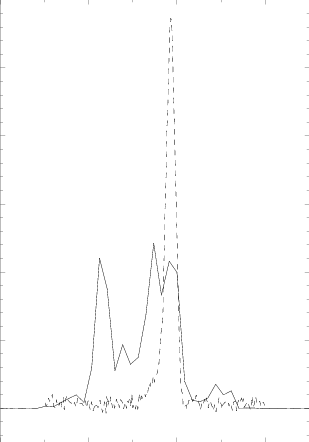
As previously mentioned, astrophysical masers form due to certain temperatures, pressures and the size of the gas producing the maser. This means that the presence of masers also allow scientists to guess the environment present in parts of the objects they study.
Astrophysical masers due to comets
There are objects in our solar system that create astrophysical masers. One such object was the planet Jupiter after it’s impact with the fragments of comet Shoemaker-Levy 9 in 1994. This was the first time cosmic masers were detected in our solar system.
Analysis of three of the impact sites on Jupiter reveal maser emissions at a frequency of 22 GHz. Such frequency can only be produced by water molecules that have been excited by thermal energy from the impacts.
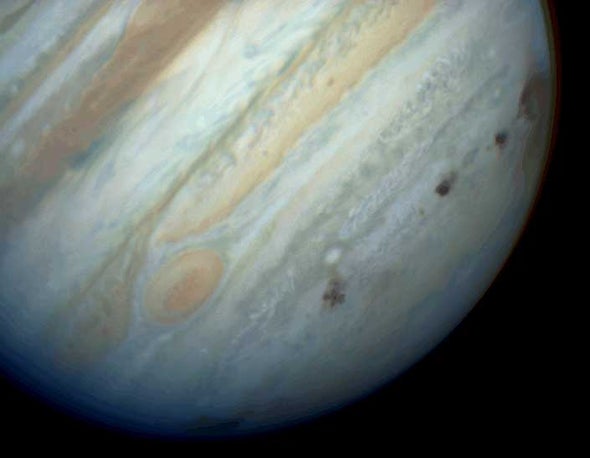
According to some scientists, masers such as these could be used for detecting water on exoplanets in other solar systems.
Astrophysical masers have also been detected from comets themselves. One such example is the comet Hale-Bopp.
On Hale-Bopp, heat from the sun vaporizes water molecules off it’s surface. Radiation from the sun then breaks the water into OH radicals. More radiation energizes these radicals and cause them to emit maser radiation at 1665 MHz and 1667 MHz frequencies.
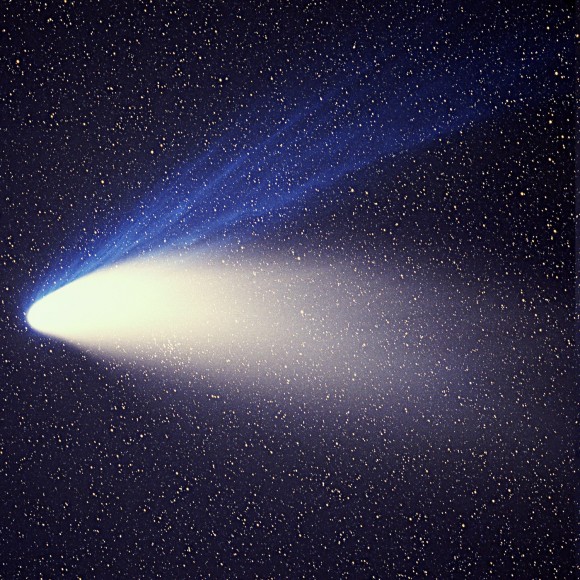
Cosmic masers and the presence of atmospheric water
Planets and comets are not the only things in the solar system to host masers.
As it turns out, Saturn’s moons Enceladus, Titan, Atlas, and Hyperion also have masers. These masers have a frequency of 22 GHz and are thus due to water molecules. This should not be surprising as water vapor plumes have been found by Cassini’s Ultraviolet Imaging Spectrometer.
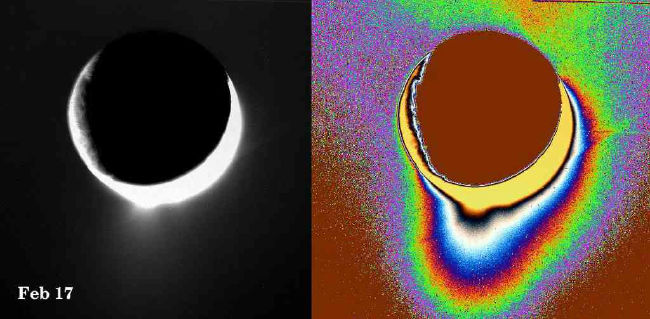
There is still debate as to what causes the maser emissions on Saturn’s moons. Most scientists think that collisions between water molecules energized them and created a population inversion.
However, others think that interactions with solar wind plasma, Saturn’s magnetic field, or shocks generated by the solar wind could also play apart.
Like the previous example, masers like these can be used to detect the existence of water on exoplanets and exomoons. Their highly amplified nature along with the distinct shape they make in their spectrum makes it easy to identify them from far away.
Astrophysical masers in star forming regions
Masers can exist inside the atmospheres of stars, nebula, and supernova remnants interacting with molecular clouds. The existence of masers in these objects has help astronomers gather more information about them.
The masers in star forming regions have allowed scientists to study how the movement of gas in molecular clouds form stars. It has also allowed scientists to study how such gases affect stars in their early lives.
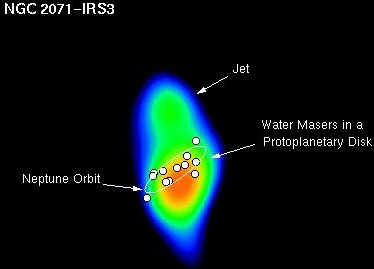
By analyzing the red and blue shifts of maser radiation created by hydrogen recombination, scientists were able to determine the shape and properties of the disk of material around the young massive star MonR2-IRS2. They were also able to confirm the existence of a fast ionized wind coming from the star.
Mapping the Milky Way with cosmic masers
As previously stated, maser radiation is highly amplified. On top of that, dust and gas scattered throughout the Milky Way do not block microwave radiation. These properties make astrophysical masers great for studying objects on the far side of the Milky Way.
With the ability to take advantage of these properties, scientists used these objects to create a map of their positions along with their velocities. This allows scientists to study how objects move around the Milky Way. It also allowed scientists to discover that our own solar system is orbiting faster and closer to the Milky Way’s center than previously thought.
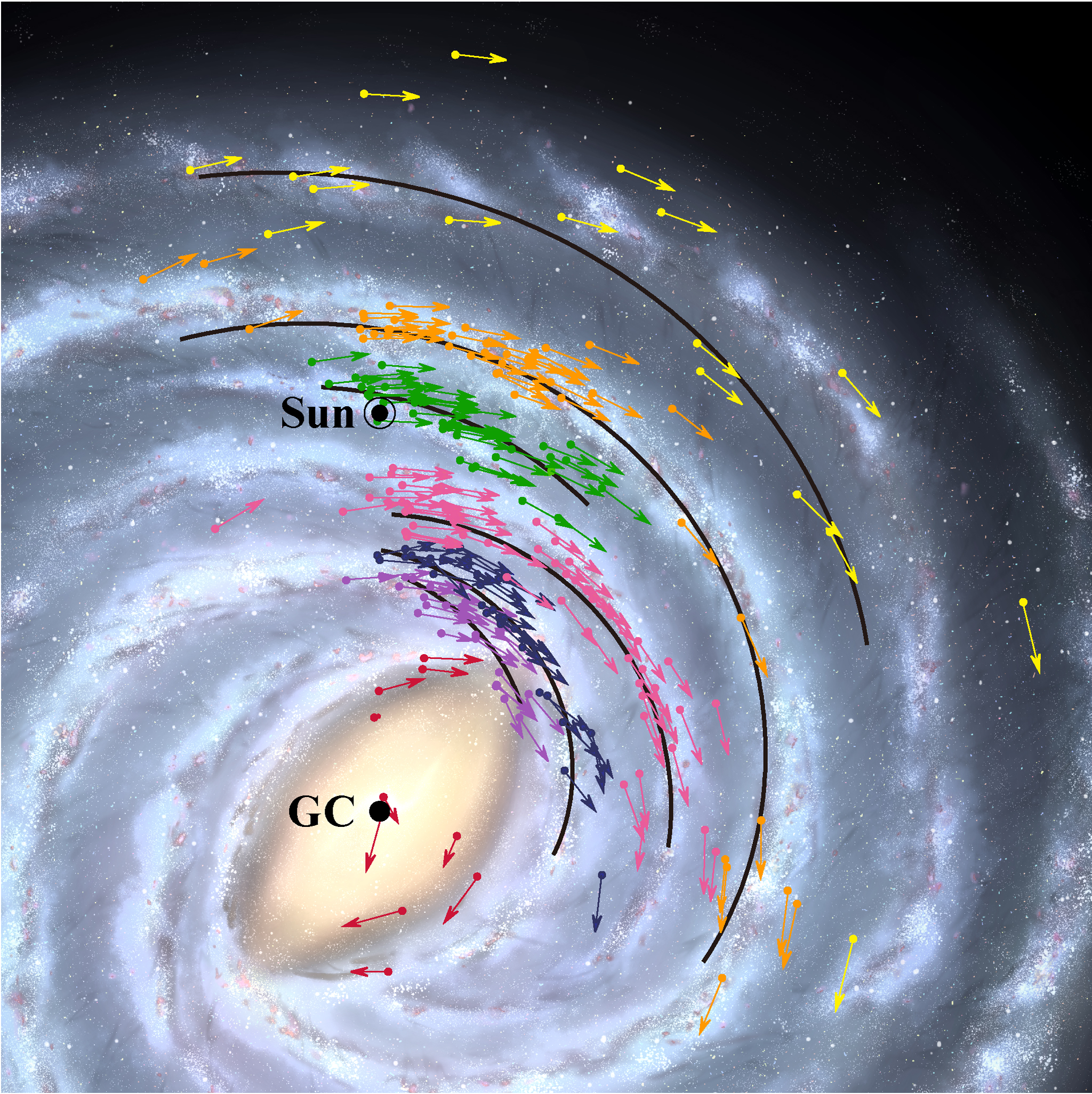
Astrophysical masers in old stars
Oxygen rich stars with low surface temperatures have maser emissions in their atmospheres. Such stars include asymptotic giant branch (AGB) stars.
These stars are so big that they don’t hold on to their atmospheres very well. On top of that, they experience pulsations caused by unstable nuclear fusion in their cores.
This serves to slowly eject the star’s outer layers into space forming a cloud of material around it.
Shockwaves from pulsations in the star’s core act as a pumping mechanism causing population inversion which results in maser emissions from silicon oxide, hydroxyl, and water molecules.
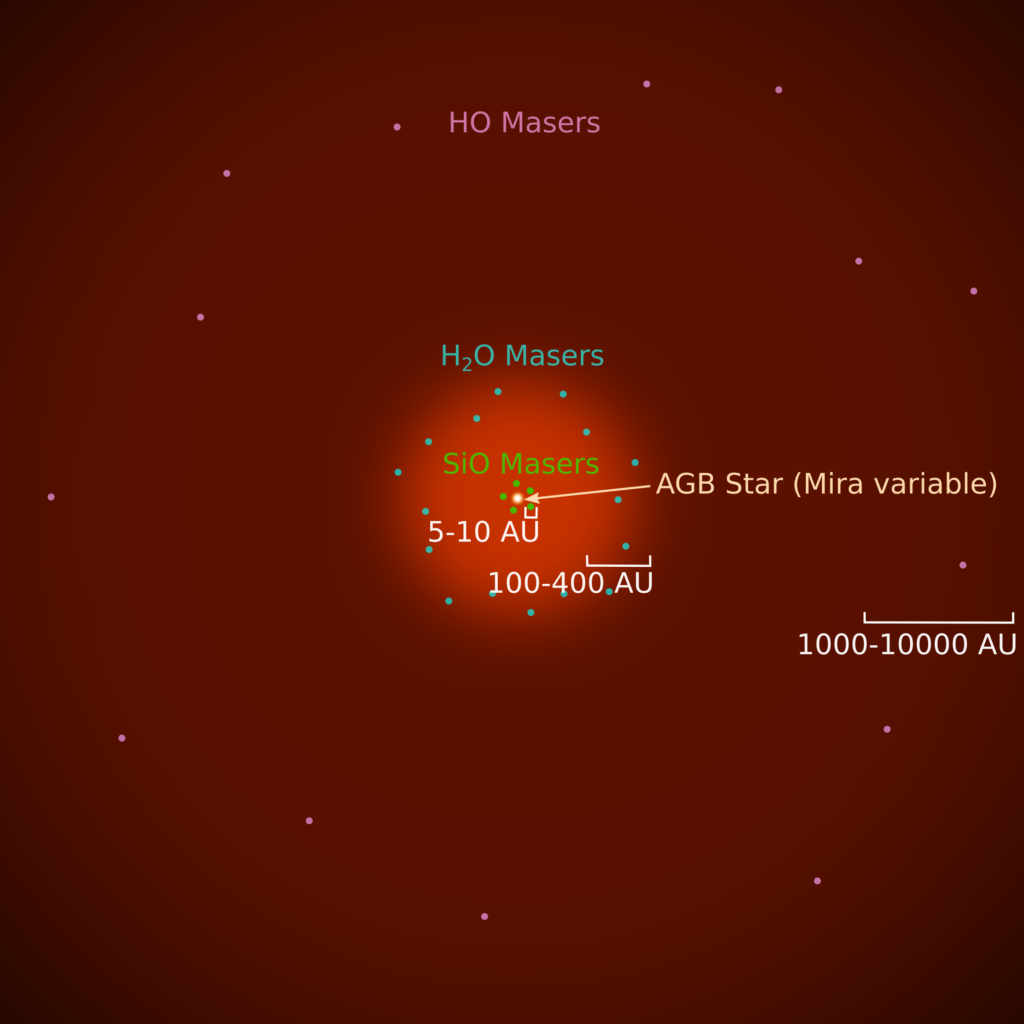
Megamasers
The most luminous astrophysical masers are thousands of times brighter than the Sun. We call these megamasers. Most of them are outside our Milky Way.
These megamasers are hundreds of millions of times brighter than cosmic masers found within our galaxy. Most of them are hydroxyl masers. Although others can be water, formaldehyde, or methane masers.
Hydroxyl megamasers are often due to high rates of star formation in luminous infrared galaxies. Such galaxies become what they are due to collisions with other galaxies. These collisions cause gas clouds to become unstable and collapse leading to mass star formation.
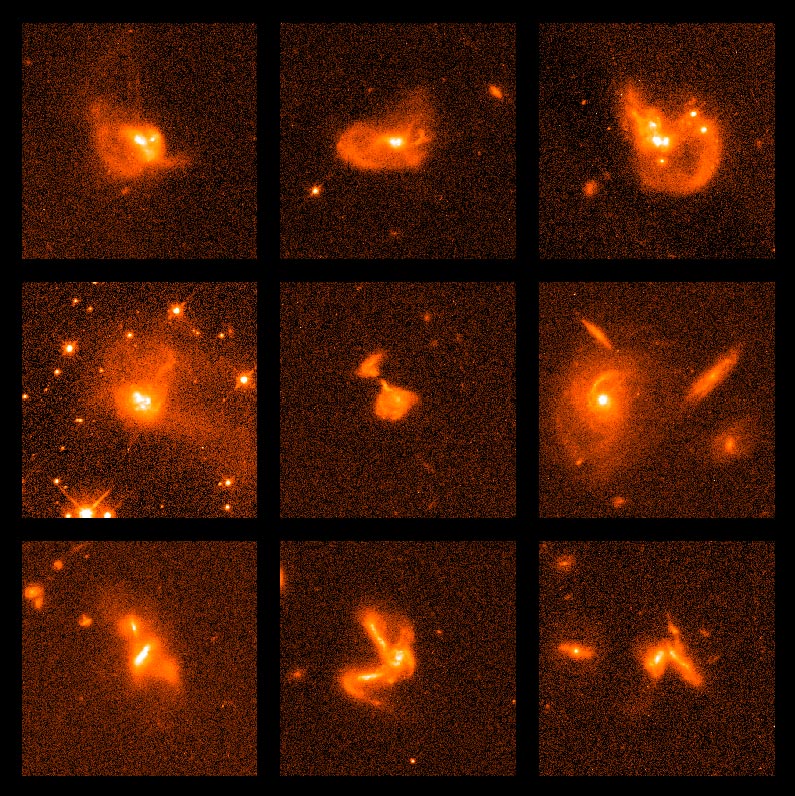
Water Megamasers are often around the center of active galactic nuclei. Material near the center of these galaxies fall into their supermassive black holes. As they do so, most of the material settles into a fast spinning disk.
In the disk, shockwaves travel throughout and act as pumping mechanisms imparting energy into water molecules. This results in population inversion.
Radiation from hotter parts of the disk cause stimulated emission in these water molecules creating bright astrophysical masers.
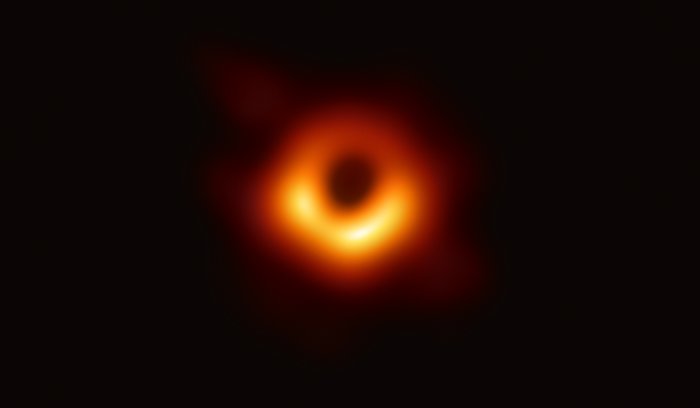
Using megamasers to find the earliest signs of water
The luminosity of megamasers has allowed scientists to look at distant objects and find out if water was present in the early universe.
This happened with MG J0414+05534; a distant quasar that is 11.1 billion light years from Earth. At that distance, the quasar appears to us as it was when the universe was only 2.5 billion years old.
MG J0414+05534 is located behind a massive galaxy that is closer to us. This is a good thing as the gravity of the massive galaxy causes a gravitational lensing effect that bends the quasar’s light and magnify it. This makes MG J0414+05534 easier to see and study.
The detection of a water megamaser from the quasar by Impellizzeri et. al. 2008 confirmed that water was already present in the universe when it was only 2.5 billion years old.
By that point, stars would have produced enough oxygen for the existence of water.
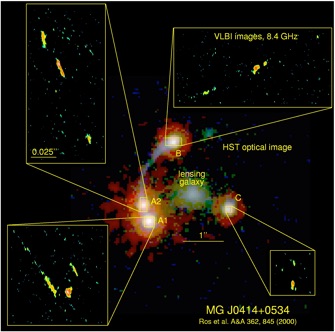
Measuring distances with megamasers
When studying the centers of active galactic nuclei, we can measure the red and blue shifts of megamasers in the disks around their super massive black holes.
This gives us the velocities of those masers and how they change over time. With this information, we can find the gravitational acceleration that keeps these masers in their orbits.
With this acceleration, velocity and the angular separation between the maser and the center of the disk known, we can find the distance between the AGN and Earth.
Using this distance, we can find the actual radius of the maser’s orbit around the center of the disk. This along with the velocity of the maser allows us to find the mass of the object at the center of the accretion disks of multiple AGNs and confirm that they are indeed supermassive black holes.
One particular galaxy that this method has been applied to was NGC 4258.
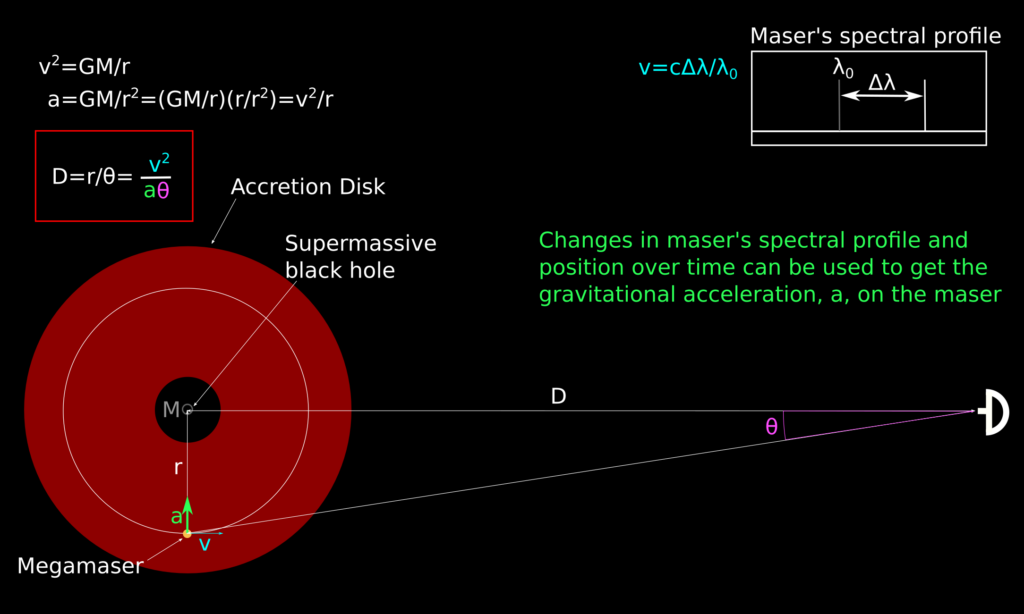
The great thing about this method is that it does not rely on the distance ladder where errors in previous methods would affect the results. On the contrary, using megamasers to measure distances could be used in correcting those methods improving their accuracy on intergalactic scales.
Finding the Hubble constant with megamasers
There is one other benefit of using megamasers to find distances. That is that it can be used to find Hubble’s constant. This was demonstrated by Pesce et. al. 2020.
With this method, Pesce’s team got a Hubble constant of 73.9±3.0 km/s/Mpc. This is almost the same as the value obtained with earlier methods using cephid variables and type Ia supernova. However, it is different from the one obtain using the Planck CMB data. That last method gave a Hubble constant of 66.9±0.6 km/s/Mpc.
As a result, this unfortunately adds more mystery to the current “Hubble tension” or “Crisis in Cosmology”.
Previously, it was possible that the Hubble constant obtained from type Ia supernovae may not be accurate. This was because of the possibility of incorrect assumptions in the nature of type Ia supernovae. Now, the results from the megamaser distance method has dispelled that idea making the discrepancy between the two results likely to be real.
Conclusions
Astrophysical masers are an example of how nature could produce coherent and amplified radiation with extremely narrow frequencies.
As the examples in this article has shown, this makes them very helpful to scientists when studying other objects and to uncover the mysteries of the universe.
Over to you now. What other things do you know about astrophysical masers and megamasers that interests you?
Also, if you like this article, please share it.
References
Masers – SAO Encyclopedia of Astronomy
Water masers on Saturn;s moons
Dark energy and the Hubble constant
Masers detect at impact site after collision between Shoemaker-Levy 9 and Jupiter
Water maser in protoplanetary disk
Masers on the far side of the Milky Way