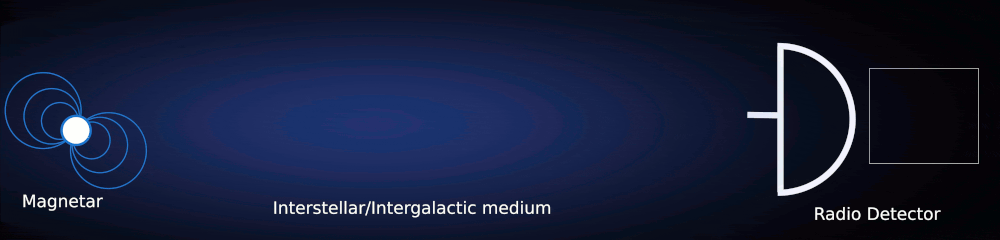
Introduction
Astronomers are trying to find new ways to solve the Hubble Tension (also called the Crisis in Cosmology). One of these ways is measuring the Hubble constant with magnetars.
Magnetars are neutron stars with extremely powerful magnetic fields. In some magnetars, these magnetic fields can cause bursts of radio waves from time to time.
Scientists have shown that these bursts are responsible for some of the mysterious fast radio bursts.
These radio waves pass through plasma in interstellar and intergalactic space. This causes certain effects on their speed.
It turns out that by studying the degree of these effects, we can know how far the waves travelled. Combine this with the redshift of the object and we get the Hubble constant.
This was what the team of scientists who wrote the paper Hagstotz et al., 2021 did.
The Hubble constant and Crisis in Cosmology
The Hubble constant relates the speed at which a galaxy moves away from an observer to it’s distance from that observer. This moving away is due to the expansion of the universe.
In terms of the big picture, the Hubble constant indicates how fast the universe is expanding and how old it is. Consequently, this mere constant is related to some very important aspects of the Universe.
The oldest way of measuring the Hubble constant is using standard candles such as cephid variables and type Ia supernova.
Standard candles are sources of light with highly predictable brightness. This allows us to compare their apparent brightness with what we think is their actual brightness. From this, we can know how far away the object is.
This method (also known as the distance ladder method) gave a Hubble constant of around 73 km/s/Mpc.
There is another way we can measure the Hubble constant. This time by using the distances between the speckles in the map of the Cosmic Microwave Background.
Early studies with this method gave a result that is nearly the same as the distance ladder. However with the high resolution image from the Planck satellite, this method now gives a Hubble constant a value of around 67 km/s/Mpc.
This new value is significantly different from the distance ladder result. This is the Hubble Tension or Crisis in Cosmology.
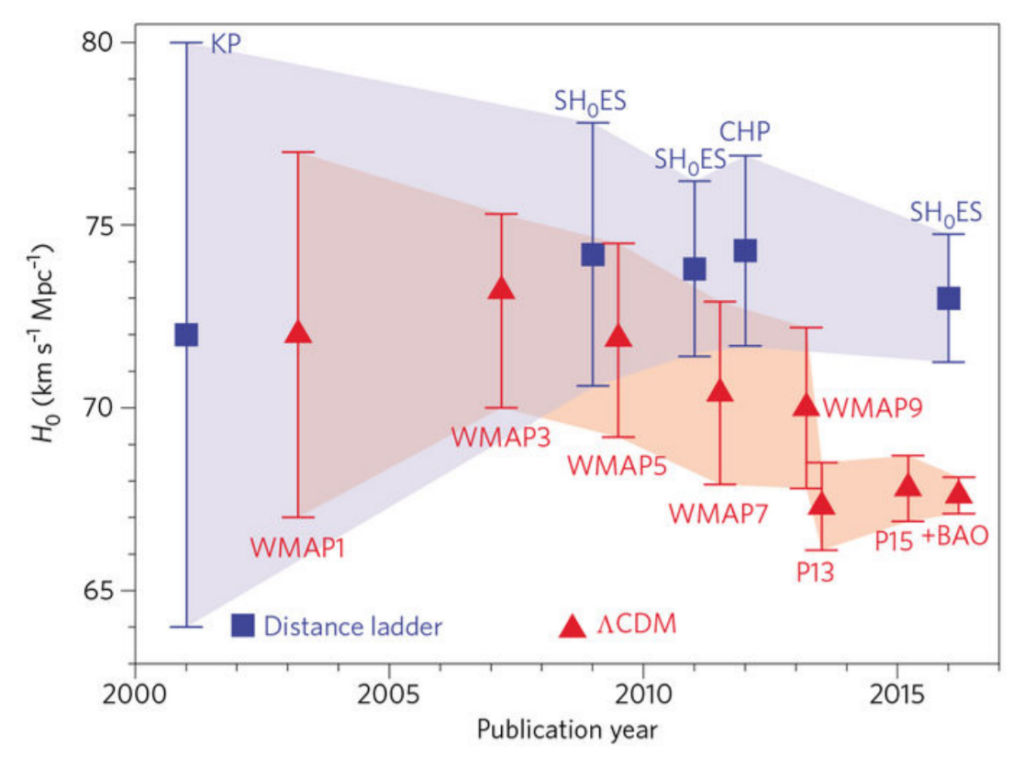
The existence of this disagreement could be a hint that we may be incorrect on certain things about the universe.
Magnetars
Since the discovery of the discrepancy in the Hubble constant between the Planck data and distance ladder method, scientists have used other ways to find the Hubble constant.
The reason to use these methods is that their accuracy isn’t dependent on using other methods of measuring distance for calibration. This is not the case with the standard candles in which errors in assumptions or calibrations could make them unreliable.
One of these alternative methods is to measure the distances of magnetars based on properties of the radio waves they give off during a fast radio burst (FRB).
Magnetars are neutron stars with extremely powerful magnetic fields. Like other neutron stars, magnetars formed from the deaths of massive stars between 8 to 20 times the mass of the Sun.
At this time, we don’t exactly know why some neutron stars become magnetars. What we know is that magnetars have magnetic fields that are so powerful they can literally disintegrate molecules in humans turning them into a cloud of atoms.
We think that the magnetic field of magnetars are so strong that strange quantum effects start to occur.
One of these effects is creating electron and positron pairs out of the vacuum. These electrons and positrons then gyrate around the magnetic field at high speeds. The result is that they produce powerful radio waves.
These manifests as periods of outbursts from the magnetar. The radio waves produced by these outbursts are so bright that they can be detected in other galaxies.
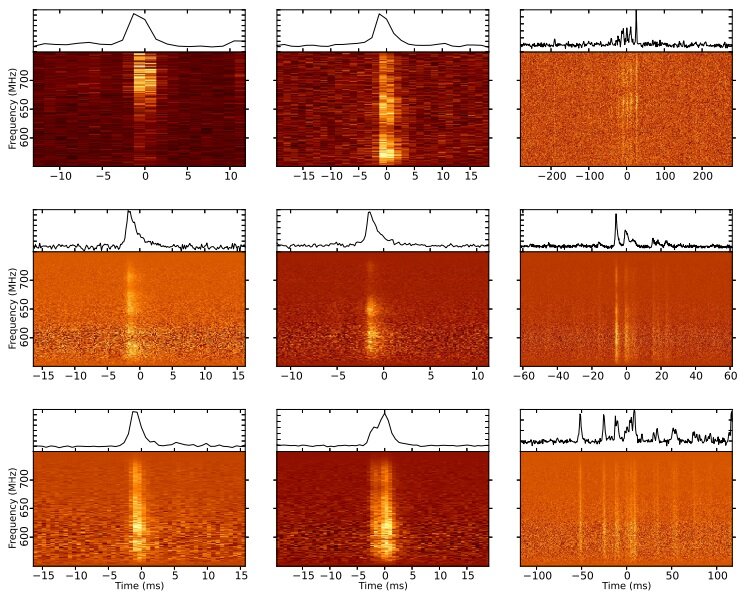
It turns out that magnetar outbursts are responsible for some fast radio bursts. This is important for measuring the Hubble constant with magnetars.
How measuring the Hubble constant with magnetars work
We can measure how far a magnetar is from Earth based on the radio waves it emits during an FRB. We can do this by measuring the delay in arrival of the magnetar’s radio waves across it’s frequency range.
This delay is because space is full of plasma which consists of atomic nuclei and electrons not bond to any atom. These free electrons absorb and emit photons including radio waves.
The rate at which they do this depends on the frequency of the photon. Lower frequency radio photons are more affected by this than higher frequency photons.
During a fast radio burst, magnetars emit radio waves at a range of frequencies all nearly at the same time.
As the waves pass through the interstellar and intergalactic medium, lower frequency photons start to lag behind the higher frequency photons due to interactions with the free electrons. The further the waves travel, the more this lag increases.
The result is that the waves reach the detector smeared out across time. By measuring the degree of smearing out, we get the amount of electrons that the photons pass through before reaching the detector.
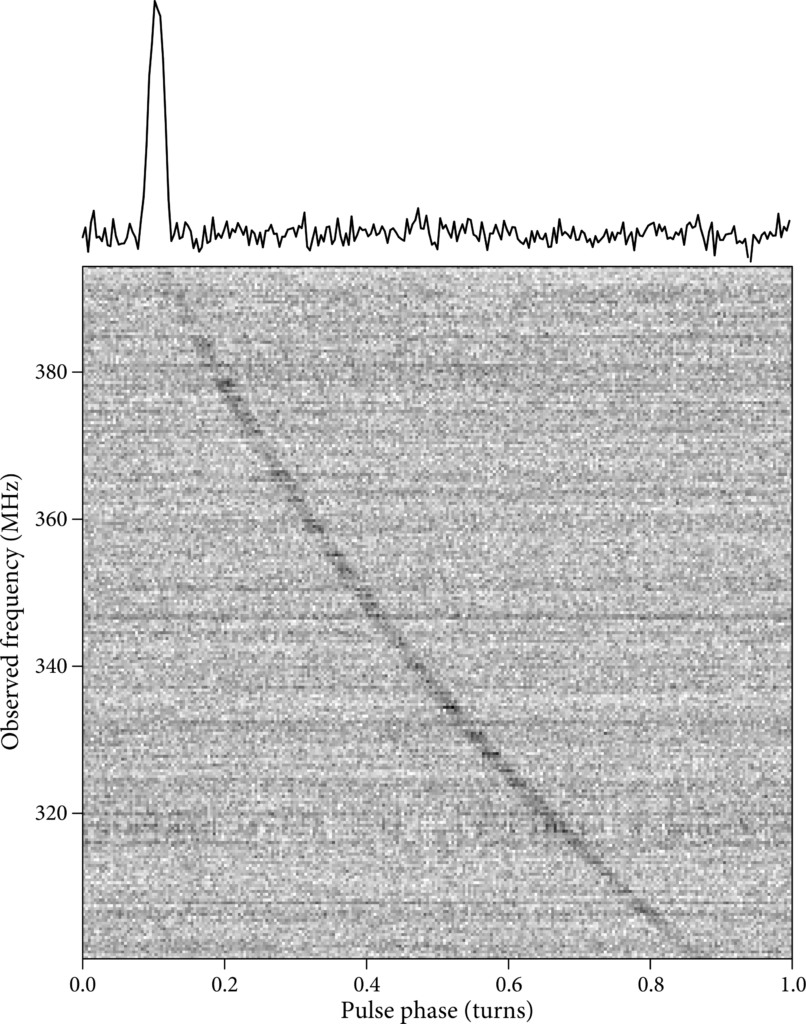
If we know the density of free electrons along the path between the Earth and the magnetar, we can get the magnetar’s distance. With the distance, we can get Hubble’s constant.
This was what Hagstotz et al., 2021 did.
As previously mentioned, the great advantage of this method is that it does not rely on the distance ladder. Neither does it rely on the method which uses the Cosmic Microwave Background.
Results from measuring the Hubble constant with magnetars and it’s implications
Hagstotz’s team used a total of nine FRBs in their study. The result they got was a Hubble constant of 62.3±9.1 km/s/Mpc.
This is a lower value than the Hubble constants from both the Planck CMB data (67 km/s/Mpc) and the distance ladder method (73 km/s/Mpc).
Before taking this to be the result of this method, we must know that Hagstotz’s team only had nine FRBs to work with. This is not very much.
Furthermore, three of the FRBs in their sample did not have their hosts identified. Thus it is unknown if those three FRBs came from magnetars.
Nevertheless, Hagstotz’s team showed that taking away these three FRBs did not change the result significantly. However, the fact still remains that more observations of FRBs need to be taken.
The value from measuring the Hubble constant with magnetars could be similar to the one from the Planck data. If that is the case, it could indicate that there are errors in the distance ladder methods.
The author of this post thinks that it is more likely that the result would be closer to that of the distance ladder method. The reason for this is that another method that uses megamasers has given nearly the same value as the distance ladder method.
If this happens, it could reinforce the idea that our understanding of cosmology may not be as correct as we thought.
Current limitations with measuring the Hubble constant with magnetars
As previously stated, one of the limitations to the study by Hagstotz et al., 2021 is that it only has a sample of 9 FRBs. As time passes, Hagstotz’s team expects this sample to increase as more FRBs from magnetars are identified.
The problem is that such objects appear to be rare. This means the rate at which we find more magnetars will be slow. Getting a better measurement of the Hubble constant may take awhile.
Conclusion
The method of measuring the Hubble constant with magnetars will undoubtedly help us uncover more about the universe. For now though, this method isn’t accurate as astronomers have only identified a small number of FRB producing magnetars.
According to Hagstotz’s team, this number will increase as future sky surveys find more of them.
Having large numbers of FRB producing magnetars to study may even lead to the discovery of any deviations in some fundamental theories such as Einstein’s General Relativity.
Over to you now. Do you think that measuring the Hubble constant with magnetars will give the same value as the Planck data or the distance ladder? If so, what do you think the implications will be? Comment down below.
Also, if you like this article, please share it with others and consider subscribing to our email list for alerts on new articles.